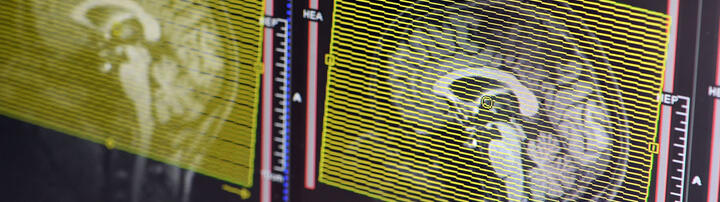
Niendorf Lab
Experimental Ultrahigh-Field MR
Profile
Experimental Ultrahigh Field Magnetic Resonance
Head: Thoralf Niendorf
Mission Statement
Alleviating the increasing incidence of the deadliest diseases requires the pursuit of multiple strategies for detection, risk assessment, treatment and follow-up. This in turn necessitates new ways to visualize complex biological systems and to image disease states at several scales of length and time, and to translate these research findings into practical clinical applications with real impact. These challenges motivate our research which concentrates on magnetic resonance (MR), to enable new ways of mapping morphology, function, physiology and metabolism. We explore the benefits of ultrahigh field (UHF) MR, as “image-makers who peer deeper and deeper into healthy and diseased tissue” (mdc-berlin.de/news/news/thoralf-niendorf-image-maker). These efforts aim to characterize (patho)physiological mechanisms and biophysical processes to integrate basic research into clinical science. We are driven to link imaging findings with underlying biological meaning, to translate what we see mesoscopically and microscopically into molecular and biophysical terms. This requires synergies between imaging and data science, leveraging artificial intelligence (AI) to bring our results to clinical translation. This is also why our research is not bound to one organ or disease; solutions to problems in one organ or disease can often be applied to challenges posed in other contexts. For this purpose we build on an open and interdisciplinary perspective, to promote strong ties across imaging modalities and systems, and to connect views across length and time scales with mechanistic and molecular insights, ultimately to address a major chasm in healthcare: promoting early interception of disease rather than late-stage treatment. Indeed, breaking new spatial and temporal boundaries and improving detection levels continues to catalyze our MR research, and our technological developments can give us the ability to observe biological processes that we could not see before. These innovations and their applications in basic research and clinical practice have the potential to impact patients and society, in line with the chief goal of the MDC: discovery for tomorrow’s medicine.
Rich Opportunities for Discovery
One of the most exciting areas of innovation in biomedical research concerns the visualization on multiple scales in space and time: imaging biological objects in size ranging from Ångströms and nanometers to the scale of whole body imaging, and from picoseconds to decades in large population imaging studies. In the Century of Biology, enabled by the tools of information technology and artificial intelligence, one can imagine a concept like ‘Google Maps’ for each fundamental biological research question, in which views at the macroscopic, mesoscopic, microscopic and the nanoscopic scales are stitched together for seamless zooming, depending on the needs and interests of the scientists and users, the research fields and the patients.
While many diseases stem from defects at the molecular and cellular levels, they play themselves out at the scales of major organ systems and the whole organism. One unique function of biomedical imaging is to monitor these levels simultaneously in vivo, connecting the view of physiologists and clinicians. The observation of physical quantities and the understanding of complex biological phenomena benefits from strong ties between imaging modalities and research fields, connecting enhanced acquisition techniques with novel post-processing algorithms, nanoscopic views with mesoscopic pictures and quantitative maps obtained on the tissue or organ level with mechanistic insights and macroscopic integrative (patho-)physiology to tackle four connected key research questions:
- How can biological objects reliably identified and noisy observables robustly quantified using fully integrated biomedical imaging pipelines, ranging from the source and raw data to image analysis and artificial intelligence?
- How can molecular mechanisms and pathological processes be optimally and nonâinvasively monitored, and their heterogeneity detailed and dissected with unprecedented high definition in spatial and temporal resolution by developing and applying novel acquisition, reconstruction, data analysis and computer-aided tools?
- What are the links between surrogate imaging markers, molecular profiles, biochemical markers and the signatures from physiological gold standard measurements? What can these relationships tell us about the stages and evolution of diseases over time, and responses to therapy and intervention?
- How can imaging technologies and scientific findings emerging from biomedical imaging sciences best transferred to human patients for fostering the prevention, early detection and treatment of disease?
Answering these fundamental questions is in the center of our research.
News
New images through high magnetic field strengths
New images through high magnetic field strengths
In mid-September, researchers from all over the world gathered in Berlin to explore the current and future possibilities of magnetic resonance imaging. The annual symposium was hosted by the team of physicist Professor Thoralf Niendorf from the Max Delbrück Center.
1st Prize Renal MRI trainee abstract competition 2024
1st Prize Renal MRI trainee abstract competition 2024 ISMRM & ISMRT Annual Meeting & Exhibition | 04-09 May 2024 Ehsan Tasbihi
Poster award 1st Prize 2024 ISMRM & ISMRT Annual Meeting & Exhibition | 04-09 May 2024 Faezeh Rahimi
Title: Whole Brain Coverage Multi-Target Framework for Benchmarking RF Arrays Tailored for Thermal Magnetic Resonance of Multiforme
Research Article
Title: In vivo monitoring of renal tubule volume fraction using dynamic parametric MRI
Magn Reson Med. 2024 Jun;91(6):2532-2545. doi: 10.1002/mrm.30023. Epub 2024 Feb 6. Authors. Tasbihi et al.
Title: MRI of kidney size matters
MAGMA 2024 Jul 3. doi: 10.1007/s10334-024-01168-5. Online ahead of print. Authors. Niendorf et al.
Title: Dynamic parametric MRI and deep learning: Unveiling renal pathophysiology through accurate kidney size quantification
NMR Biomed . 2024 Apr;37(4):e5075. doi: 10.1002/nbm.5075. Epub 2023 Dec 3. Authors. Klein et al.
Title: Monitoring kidney size to interpret MRI-based assessment
of renal oxygenation in acute pathophysiological scenarios
Title: Reliable kidney size determination by magnetic resonance imaging in pathophysiological settings
Acta Physiol 233 (2): e13701 DOI: 10.1111/apha.13701 Autors: Gladytz et al.
For further details about resources and projects please visit https://buffportal.mdc-berlin.de.
Berlin Ultrahigh Field Facility
Events
Team
Research Areas
Mechanistic Focus: Understanding Renal (Patho)Physiology and Fighting Kidney Disease
Wordwide, the role of kidney health is overlooked in longevity and quality of life. Hypoxia-driven acute and chronic kidney diseases pose a significant worldwide socio-economic burden on healthcare. The kidney is a remarkable organ. Unlike other organs, blood supply in the kidney drives O2-consuming tubular reabsorption and determines kidney function. The unique relationship of O2 delivery/consumption means that renal tissue partial pressure of O2 is low, putting the kidney always at the brink of hypoxia. Imagine if physicians could use renal oxygenation and hypoxia with relevant diagnostic and prognostic information, before the onset of AKI for early disease interception. We hypothesize that MRI based fingerprinting of renal oxygenation using step response analysis – a systems analysis approach which is well-established in engineering for quantitative analysis of mechanical and electronic systems - (i) facilitates clear distinction between different AKI grades, (ii) provides classifiers and markers of the functional states of the kidney that allow early diagnosis and prognostic risk assessment of AKI and (iii) suits swift translation to human application owing to the non-invasive nature of MRI. Our developments have been met with great interest from clinical partners including our contributions to consensus-based technical recommendations for clinical MRI of the kidney, which has the potential to impact patients and society.
Figure 1: Multi-parametric renal MRI for probing different physiological parameters at different spatial scales. Coronal views of rat kidneys: a) photograph of freshly excised kidney, b) T1-weighted anatomical MR microscopy acquired ex vivo using an in-plane spatial resolution of 50 mm (cortex COR; outer medulla OM; inner medulla IM), c) T2* sensitized MR image acquired ex vivo using an in-plane spatial resolution of 50 mm, d) Mesoscopic in vivo parametric map of the MRI relaxation time T2 which represents the blood oxygenation level at the more capillary level (in-plane spatial resolution 300 mm), e) In vivo map of the MRI relaxation parameter T2* (in-plane spatial resolution of 300 mm) which is a surrogate for blood oxygenation of large venous vessels, f) Apparent water diffusion coefficient (ADC) map with a spatial resolution of 300 mm showing Brownian water motion at the scale of 50 mm, g) In vivo map of renal cortical and outer medullary renal blood volume fraction (BVf) obtained from T2* mapping using an intravascular contrast agent (4 mg Fe/kg USPIO). The 1 cm scale bar illustrates the size of the rat kidney.
ERC Advanced Grant: Thermal Magnetic Resonance to Study the Role of Temperature in Biology and Disease
We focus on pioneering novel MR applications, exemplified by our push towards Thermal Magnetic Resonance (ThermalMR) to study the role of temperature in biology and disease. Our group developed the concept of thermal phenotyping. For this project, Thoralf Niendorf was awarded an ERC Advanced Grant. To master the physics, we performed numerical electromagnetic field (EMF) simulations and developed RF applicator hardware concepts to investigate the capacity of ThermalMR for targeted thermal treatment of deep-seated brain tumours (Figure 1). We devised algorithms for thermal dose and safety management for ThermalMR treatment of glioblastoma patients. For hardware implementation, we developed the first 32-channel modular signal source for RF heating and MRI and a multi-channel RF signal supervision module that monitors and regulates RF signals and detects patient. We demonstrated the feasibility of ThermalMR for controlled release of a model therapeutic from thermoresponsive nanogels en route to localized drug delivery.
Figure 1: ERC advanced grant project: Studying the role of temperature in biological systems and disease. Our group explores innovative MR applications that are not yet in the clinical mainstream. We developed a novel instrument for Thermal Magnetic Resonance (a,b) which supports targeted RF heating at 7.0 T for adjunct anti-cancer therapy or for release of a thermoresponsive therapeutic cargo from a nanoparticle carrier.
Technology Focus: Development of Enabling Hardware for Ultrahigh Field MR
The enhanced sensitivity afforded by UHF-MR is a driving force behind our technological developments. We pioneered multi-channel RF coil technology for cardiovascular, renal, brain and ophthalmic MRI at 7.0T. Beyond conventional 1H MR, we are exploring MRI of sodium (23Na), fluorine (19F), potassium (39K), chlorine and other nuclei for better insight into physiometabolic processes (Figure 1). We were the first to measure 39K in the human heart in vivo. We demonstrated sub-millimeter resolution 23Na MRI and microstructural probing with DWI of the human eye. This work is now ready for clinical studies in patients with ocular melanoma.
Figure 1: Enabling RF technology for Ultrahigh Field Magnetic Resonance. Novel RF coil technology including (a) wide-band self-grounded bow tie (SGBT) antenna building block for 1H and 19F MRi, (b) 32-channel SGBT transceiver array designed, and clinically evaluated by our group for cardiac MR at 7.0 T. c) Four channel 23Na array which facilitated millimeter in-plane spatial resolution sodium MRI of the human heart and kidney. d) First in vivo 39K MRI of the human heart.
Experimental Focus: Quantitative Mapping and (Nano)Molecular Probing
We are recognized leaders in preclinical MRI of the heart and kidney. Capitalizing on this expertise, we detected microvascular dysfunction in a mouse model of HCM. These findings are paramount for understanding our clinical observations. We applied acute pathophysiological interventions to rats directly within the MR scanner, probing renal hemodynamics and oxygenation using parametric MRI, to better understand the mechanisms underlying acute kidney injury (AKI). Using our integrated multi-modality setup (MR-PHYSIOL-NIRS) we demonstrated that measuring kidney size is essential for correctly interpreting acute changes in renal tissue oxygenation derived from T2*,T2. Our proof-of-principle studies suggest that physiological systems analysis of the kidney using high temporal resolution T2* monitoring of the oxygenation step response may provide a fingerprint of the kidney’s functional state, which opens an entirely new research field. We advanced the study of brain inflammation, showing transient enlargement of brain ventricles in the EAE model and in multiple sclerosis (MS) patients. We demonstrated the feasibility of 19F MRI to detect a fluorinated drug indicated in MS, teriflunomide, in the healthy and inflammed brain (Figure 1). To push the boundaries of fMRI in small rodents we developed a setup for normothermic fMRI to probe nociception, identifying a link between blood pressure changes and the murine neuromatrix (Figure 1). This led to translational experiments to address fundamental questions from perception of pain to the nature of consciousness. We addressed a key challenge of murine fMRI: maintaining functional brain states approximating human volunteers. We contributed to a consensus of fMRI connectivity analysis in the rat brain which was published in Nature Neuroscience.
Figure 1: Experimental MRI and Preclinical Applications: Quantiative Mapping and (Nano)Molecular Probing. a) Understanding the mechanisms of acute kidney injury (AKI) is our motivation for MR assessment of renal haemodynamics and oxygenation. b) First In vivo 19F MR detection of the MS drug siponimod in the stomach. c) 19F MR image of an ex vivo EAE mouse acquired at 21.1 T using a of resolution 153 µm³, and d) We contributed to the developemt of a reproducible pipeline to accommodate rat fMRI data acquired with various protocols and determined experimental and processing parameters associated with greater connectivity detection.
Focus on Data Science and Machine Learning (ML)
Obtaining a mechanistic understanding of processes for early disease interception requires that we use data science to integrate insights from MRI with analytical appraoaches and biophysical models (Figure 1). We established a biophysical model to estimate changes in O2 saturation of haemoglobin from changes in T2* and kidney size. For this purpose we developed a geometry-based model to assess changes in kidney size, facilitating accurate and precise measurements equivalent to manual segmentation but >70-fold faster. To enhance speed even further, we developed a deep dilated U-Net (DDU-Net) and demonstrated its superior speed and performance versus a self-configuring U-Net (Figure 1). This allows us to move beyond retrospective kidney size assessment, towards prospective real-time application by directly integrating DDU-Net into the MR scanner’s reconstruction pipeline. In another project we simulated rigid head motion artifacts on large-scale epidemiological MRI datasets (e.g. NAKO) and evaluated the impact on automatic ML brain segmentation (Figure 1). We evaluated MR contrast-agnostic ML brain segmentation tools on heterogeneous clinical datasets to ensure robustness of our clinical findings on brain ventricle size changes in pediatric patients. We contributed to ML studies focusing on brain age modeling volume and topography of adipose tissue in the body trunk, vessel segmentation in cerebrovascular disease or on abdominal segmentation (Figure 1).
Figure 1: Achievements in data science and machine learning. a) Monitoring kidney size allows physiological interpretation of acute renal oxygenation changes obtained by a biophysical model driven MRI-oximetry. b) ML based segmentation of the kidney from MRI, in order to use kidney size as a (pre)clinical biomarker for renal disease is potentially instrumental for developing MRI-based diagnostic tools for kidney disorders and for gaining new insight into renal pathophysiology. c) ML based segmentation revealed that pediatric patients with acute disseminated encephalomyelitis have enlarged brain ventricles. d) Assessment of volume and regional spatial distribution of fat compartments of the body trunk using deep learning in 11 141 participants of the German National Cohort.
Publications
News
Jobs
Interested candidates may send CV and cover letter to
thoralf.niendorf@mdc-berlin.de.
Alternatively, you can directly apply to one of the following research programs at the B.U.F.F. that recently announced open positions. Please mind the application details in the projects' description.
–––––––––––––––––––––––––––––––––––––––––––––––––––––––––––––––––––––––––––––––––
Post-Doc Positions in Ultrahigh Field Magnetic Resonance
The Berlin Ultrahigh Field Facility (B.U.F.F.) at the Max Delbrück Center for Molecular Medicine (MDC) in the Helmholtz Association, Berlin, Germany is seeking enterprising scientists interested in the development of radiofrequency technology and imaging methodology tailored for ultrahigh field magnetic resonance (UHF-MR).
PhD Positions in Ultrahigh Field Magnetic Resonance
The Berlin Ultrahigh Field Facility (B.U.F.F.) at the Max Delbrück Center for Molecular Medicine (MDC) in the Helmholtz Association, Berlin, Germany is seeking enterprising scientists interested in the development of radiofrequency technology and imaging methodology tailored for ultrahigh field magnetic resonance (UHF-MR).
Translational imaging of brain function
How does the brain work? How does external input impinge on internal brain states? How did the functional underpinnings emerge throughout evolution?
In this cutting-edge research program we apply functional magnetic resonance imaging (fMRI) and complementary techniques to detail brain functions and network dynamics in humans and small animal models, with a strong clinical focus.
Contact: translational.fMRI@mdc-berlin.de
- Application details
- We currently offer Master and Bachelor thesis projects for a running period of 4-12 months. We are looking for ambitious students with an independent working style, who are keen to learn and grow in an international research environment at the interface of (f)MRI and molecular medicine. [click for details]
Application criteria Applicants should ideally hold a background in either psychology, medical or life sciences, (bio)physics, signal processing, statistics, or related fields. Background in fMRI is beneficial, but not required.
Project scope You will learn how to plan and conduct fMRI experiments in humans and/or mice, how to handle and adjust advanced functional MRI protocols, as well as complementary techniques.
Project structure Besides a classical project structure, we provide the opportunity for tandem projects in which two students collaborate and support each other. In this form individual objectives are embedded in a common research project. This makes the work more fun and efficient.
–––––––––––––––––––––––––––––––––––––––––––––––––––––––––––––––––––––––––––––––––