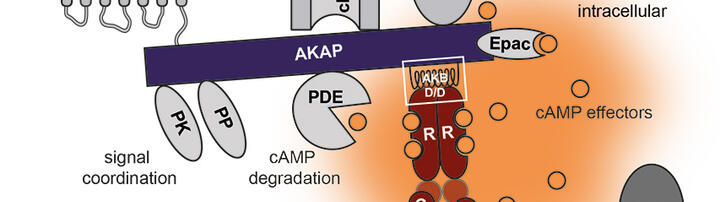
Klußmann Lab
Anchored Signalling
Profile
Cyclic adenosine monophosphate (cAMP) is a ubiquitous second messenger that has fundamental physiological functions. It regulates, amongst many others, cardiac contractility, blood pressure or body water homeostasis. To specifically control each of the different underlying mechanisms, cAMP acts in a cell type-specific spatially and temporally highly coordinated fashion. This compartmentalisation of cAMP signalling involves specific signalling proteins such as A-kinase anchoring proteins (AKAPs) and phosphodiesterases (PDEs). AKAPs are scaffolds defining the composition of cAMP signalling compartments and PDEs locally terminate cAMP signalling. Dysregulation of compartmentalised cAMP signalling can cause or is associated with diseases such as heart failure, hypertension or if water reabsorption is dysfunctional, diabetes insipidus.
Our aim is to elucidate molecular mechanisms underlying the coordination of cAMP signalling in the cardiovascular system and to reveal how its dysregulation causes disease. Based on identified signalling cascade components, we aim to identify novel targets and small molecule modulators.. This may provide starting points for new strategies towards the treatment of diseases which involve cAMP signalling compartments and for which a satisfactory treatment is not available, e.g. heart failure, hypertension, hypertension-induced end-organ injury or water balance disorders such as diabetes insipidus.
Team
Research
A plethora of extracellular cues such as hormones or neurotransmitters stimulates synthesis or release of an intracellular ubiquitous “second” messenger. Different stimuli can act simultaneously and thereby can even cause an elevation of the same second messenger at the same time. So, how does each of the different stimuli elicits a specific cellular response? It is emerging that second messengers such as the cyclic nucleotide, cyclic adenosine monophosphate (cAMP), or Ca2+ act in a spatially and temporally coordinated manner in defined cellular compartments to ensure specificity. Our group is predominantly interested in cAMP signalling compartments.
cAMP directs cellular processes predominantly by integrating inputs from G protein-coupled receptors (GPCRs), which activate the stimulatory G protein (GS). Activated GS induces adenylyl cyclases to generate cAMP. The cAMP binds and activates its downstream effector proteins, cAMP-dependent protein kinase A (PKA), exchange proteins activated by cAMP (Epac), cyclic nucleotide-gated ion channels (CNG), hyperpolarization-activated cyclic nucleotide-gated channels (HCN) and the Popeye domain containing (POPDC) proteins. Different GPCRs can, even simultaneously, stimulate cAMP synthesis in the same cell. In order to avoid random activation of cAMP effectors and to enable a specific cellular response to each stimulus, cells spatially restrict the per se freely diffusible cAMP and tether its effectors to defined cellular compartments. The latter is often achieved by A-kinase anchoring proteins (AKAPs). At specific cellular sites, AKAPs engage in direct protein-protein interactions with cAMP effectors, their substrates, and other signalling proteins such as kinases and protein phosphatases. Phosphodiesterases (PDEs) hydrolyse cAMP, are constitutively active and are strategically positioned by direct interactions with cellular compartments or through protein-protein interactions, e.g. with AKAPs. PDEs thereby locally limit cAMP levels. GS-coupled GPCRs are linked to specifically composed cAMP signalling compartments to facilitate specific cellular responses.
The Anchored Signalling group focuses on compartmentalized cAMP signalling in renal collecting duct principal cells where it controls AVP-mediated water reabsorption, and on cAMP signalling that controls blood pressure in vascular smooth muscle cells (VSMCs) and cardiac myocyte contractility. With our studies, we aim to contribute to i.) a better understanding of molecular mechanisms underlying compartmentalised cAMP signalling in the renal and cardiovascular system, ii.) to elucidating how dysregulation of compartmentalised cAMP signalling is involved in renal and cardiovascular diseases and iii.) on that basis to identify and validate novel targets for the treatment and prevention of renal and cardiovascular diseases, in particular diabetes insipidus, hypertension and hypertension-induced cardiac and kidney damage. Due to the persistent medical need for these diseases, innovative approaches for their treatment are needed.
Projects
Our projects focus on a specific PDE, PDE3A, and vasopressin-mediated water reabsorption.
Elucidating functions of PDE3A in the cardiovascular system and targeting PDE3A for protection of end-organs from hypertension-induced injury
Hypertension represents a major risk factor for secondary cardiovascular complications and diseases such as cardiac hypertrophy, heart failure, stroke, hypertensive retinopathy or chronic kidney disease (CKD). According to the WHO, an astonishing 1.3 billion people worldwide have hypertension. Worldwide, hypertension causes 8.5 million deaths from stroke, ischemic heart disease, other vascular and renal diseases annually. 64 million people worldwide are affected by heart failure. Only 50 % of heart failure patients are alive 5 years after diagnosis. These numbers reflect the burden of hypertension and hypertension-induced end-organ damage as well as the medical need for innovative and effective therapies for the prevention and treatment of hypertension and hypertension-induced end-organ damage.
Hypertension with brachydactyly type E (HTNB) is a rare autosomal dominant Mendelian syndrome so far found in only 10-20 families. HTNB is distinguished by harmless brachydactyly type E, and progressive severe hypertension resembling essential hypertension. The severe hypertension is caused by increased proliferation rates of vascular smooth muscle cells (VSMCs) and vessel hyperplasia. The systolic blood pressure of HTNB patients can reach 170-250 mmHg, their diastolic pressure 100-150 mmHg. If untreated, the patients die of stroke by age 50 years. HTNB causes vascular injury but surprisingly not the above-mentioned typical hypertension-induced end-organ damage, including cardiac hypertrophy, heart failure or kidney damage.
We demonstrated that gain-of-function phosphodiesterase (PDE)3A gene mutations cause HTNB. Through examination of HTNB patients and the study of various cell and animal models, we aim to understand the mechanisms by which the PDE3A mutations cause hypertension and at the same time protect organs from hypertension-induced injury. Elucidation of the underlying mechanisms could pave the way to novel approaches for the treatment and prevention of hypertension and hypertension-induced end-organ damage.
Vasopressin-mediated water reabsorption – trafficking of the water channel aquaporin-2 (AQP2)
Arginine-vasopressin (AVP; antidiuretic hormone) induces water reabsorption in renal collecting duct principal cells and thereby fine-tunes body water homeostasis. AVP is secreted from the hypothalamus when plasma osmolality increases, e.g. indicated by thirst. AVP binds to GS-coupled vasopressin type 2 receptors (V2R) on the basolateral plasma membrane of renal collecting duct principal cells, which leads to cAMP generation and activation of PKA. PKA initiates a signalling cascade that induces the redistribution of water channels, aquaporin (AQP)-2, from intracellular vesicles predominately into the apical plasma membrane. The insertion of AQP2 introduces water-selective pores into the plasma membrane, water from the lumen of the collecting duct enters through AQP2 along an osmotic gradient and exits the cells through AQP3 and AQP4. The latter two aquaporins constitutively reside in the basolateral plasma membrane. The plasma membrane insertion of AQP2 reduces urine output and decreases plasma osmolality; dysregulation of the plasma membrane insertion of AQP2 causes diabetes insipidus.
Diabetes insipidus is characterised by a massive loss of water, i.e. passing up to 20 l urine, per day, and thirst (polyuria and polydipsia, respectively). The water loss can cause severe side effects including seizure, hypotension and hypovolemic shock. Congenital forms of DI, caused by mutations in the genes encoding AVP, V2R or AQP2, affect around 300.000 people world-wide. Drug-induced acquired forms affect millions of patients. Lithium induces diabetes insipidus in patients with bipolar diseases (1.5 million in the US alone), and the V2R blocker, tolvaptan, induces diabetes insipidus in patients with autosomal dominant polycystic kidney disease (12.5 million people world-wide). There is no satisfactory treatment available to reduce the water loss in diabetes insipidus.
We have discovered several AKAPs and new kinases and mechanisms that participate in the cAMP-directed control of AVP-mediated water reabsorption. Moreover, we identified small molecules that promote the plasma membrane localisation of AQP2 and thus could have utility for the treatment of diabetes insipidus. Our group aims to further contribute to understanding molecular mechanisms underlying AVP-mediated water reabsorption and to develop novel small molecules towards drug candidates for the treatment of diabetes insipidus.
Funding
Our work is kindly supported by two individual grants from the Deutsche Forschungsgemeinschaft (DFG), the Else Kröner-Fresenius-Stiftung (Key project), and the MDC.
Publications
News
Miscellaneous
Dr. Saal van Zwanenbergprijs for Master student Julia Alberts
Martina Schmidt (left) and Julia Alberts (right) at the Dr. Saal van Zwanenberg Prize ceremony
On 12 November 2021 the Dr. Saal van Zwanenberg Prize ceremony took place in the Hodson House in Haarlem. Our pharmacy student Julia (M.K.) Alberts was awarded the 3rd Dr Saal van Zwanenberg Prize for excellent Master research in the field of Pharmaceutical Sciences. The Dr. Saal van Zwanenberg Prize is awarded to master students whom master project focused on the identification of drug actions and further improvements for disease treatment. Julia identified in her project that PDE3A inhibitors might act in the future as drug targets to treat cardiovascular disease. Julia aims to enlarge her pharmaceutical network with the price. In the future she aims to work in the pharmaceutical industry with focus on the improvements of regulatory affairs.
Jobs
Postdoc position
We are looking for a scientist with a PhD in a life science who is interested in translational research with a focus on the identification of drug candidates. A solid background in biochemistry and cell biology is required and background in pharmacology would be a plus.
Mutant PDE3A causes hypertension with brachydactyly (HTNB). The specific aim of this three year project is to mimic the properties of mutant PDE3A enzymes and to test whether this confers protection from hypertension-induced end-organ damage.
Open for PhD candidates
For enquires, please contact E. Klussmann and/or
apply through the MDC International PhD program. The program is open for applications twice a year.
Open for MSc and BSc candidates
We are always open for MSc and BSc candidates to carry out the work for their theses in our lab. Projects around PDE3A or the control of AQP2 are available.
For further information please contact enno.klussmann@mdc-berlin.de.